Organic Piezoelectric Biomaterials
1. Background and state-of-the-art
Organic piezoelectrics, capable to “sense” delicate forces in living organism and transfer them into effective electrical signal equivalent to the signals used by organism itself for self-regeneration, hold a promising potential for many regenerative problems currently without any solution.1 Exciting new results of Swiss clinicians recently have shown that targeted lumbosacral spinal electro-stimulation, performed by implanted device, did recover walking ability in patients with more than 4-year long spinal cord injury.2,3 They highlighted a need for very precise spatio-temporal stimulation in order to obtain permanent recovery that will work after the stimulation is stopped.2,3 However, none of the currently available materials, including the whole class of currently available piezopolymers,4-6 has any tools for controlling stimulation accuracy at cellular level. In current state of the art none of the approaches used to design organic piezoelectrics for biomedicine does not include specificity of individual patient. Personalized approach will open wide highways for organic piezoelectrics as effective solutions in medical treatments. This opens interesting novel possibilities for designing materials applicable in biomedicine, particularly in regeneration and tissue engineering.
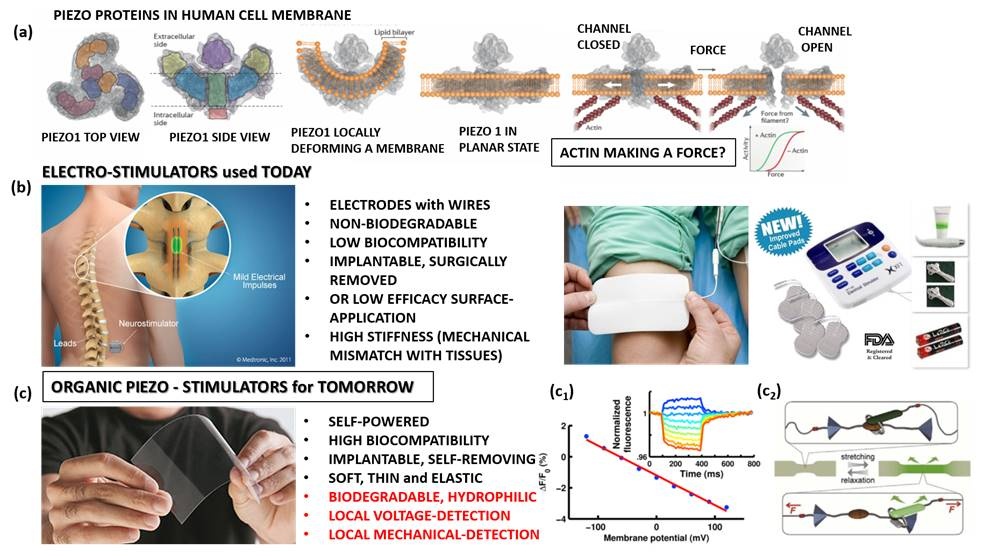
Figure 1: PIEZO proteins in a cell membrane16 (a); commercial electro-stimulators and their properties (b); piezo-stimulators, as alternative, and their future properties envisaged within our research (marked in red) (c); voltage-sensitive (c1) 17 and mechanochromic dyes (c2) 18.
2. Objectives, originality and impact on new research approaches
The main objective of proposed research is to design next generation of implantable / self-removing piezoelectric electrostimulators able to sense, detect and control electrostimulation at cellular level. For that purpose we aim:
(i) Designing novel organic piezoelectrics with hydrophilic surface, biodegradability and biocompatibility (by processing piezoelectric, surface-modified- PLLA structures),
(ii) Incorporating tools for mechano-voltage detection able to measure and emit information detected during stimulation (by incorporating mechano- and voltage- sensitive dyes into piezo-polymeric matrix),
(iii) Constructing novel piezoelectric device able to detect, heal and self-remove and (iv) exploring electrostimulation at cellular level by visualizing mechanical deformation and generated voltage.
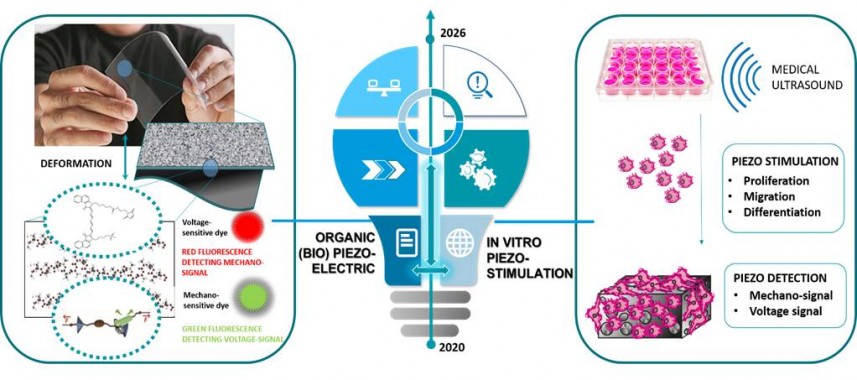
Figure 2: Illustration of the design of organic (bio)piezoelectrics and in vitro piezostimulation.
We have developed and optimized highly efficient protocol for processing piezoelectric PLLA films and modified their surface to be hydrophilic.7 Our expertise also includes surface functionalization methods like bonding small molecules (i.e. amino acids)8 and large molecules (i.e. peptides)9 on the surface of metallic nanoparticles as well as labelling bioceramics (I.e. hydroxyapatite) and polymers (i.e. PLGA) with fluorescent dyes.10 Our expertise also includes in vitro interactions of materials with bacterial and mammalian cells.11-13
3. Unique methodology
Novel method will be developed to incorporate voltage- and mechano- sensitive dyes into PLLA matrix. One of the approaches will include plasma-mediated surface group modifications that will enable covalent bonding between dyes and polymer functional groups. In addition to melting-assisted stretching, we will work on processing piezo-PLLA layers using epitaxial growth as well as 3D printing.
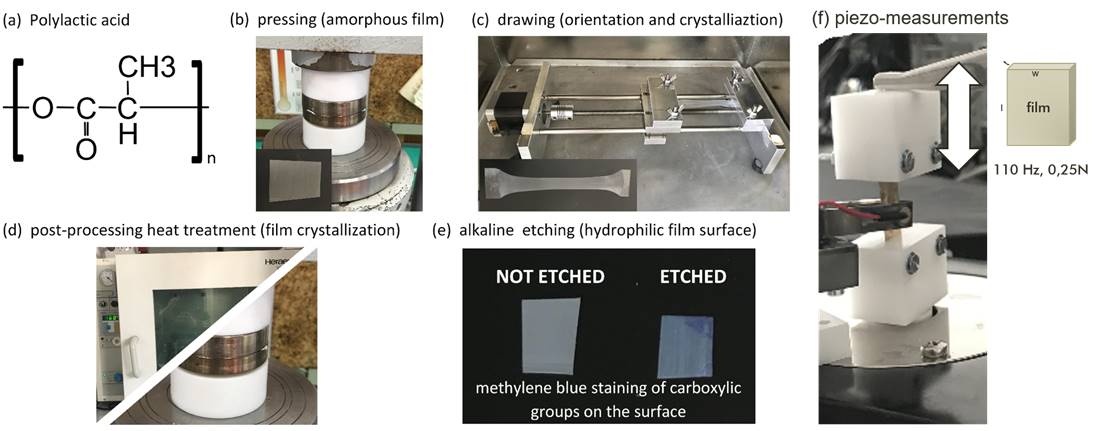
Figure 3: Hydrophilic Piezoelectric PLLA film: (a) PLLA polymer, (b) hot pressed to attain amorphous film, (c) drawn at high temperatures to impose orientation and crystallinity, (d) annealed in dryer or hot pressed at higher temperatures to enhance crystallinity and (e) alkaline etched to improve hydrophilicity of the surface which is confirmed with methylene blue staining. (by Udovc, Spreitzer, Vukomanovic; https://www.nature.com/articles/s41428-019-0281-5)
REFERENCES:
1 Meng, S., Rouabhia, M. & Zhang, Z. Applied Biomedical Engineering (ed. Gargiulo, G.) 37–62 (InTech, 2011).
2 Wagner, F. B. et al. Nature 563, 65–93 (2018);
3 Formento, E. et al. Nat. Neurosci. 21, 1728–1741 (2018).
4 Rajabi, A. H et al Acta Biomater. 24, 12–23 (2015); Ribeiroet al. Colloids Surf. B 136, 46–55 (2015).
5 Ciofani, G. & Menciassi, A. Piezoelectric Nanomaterials for Biomedical Applications. (Springer Berlin Heidelberg, 2012).
6 Ribeiro, C., Sencadas, V., Correia, D. M. & Lanceros-Méndez, S. Colloids Surf. B Biointerfaces 136, 46–55 (2015).
7 L. Udovc, M. Spreitzer, M. Vukomanovic, Polymer Journal (Nature Springer) 52, 299–311 (2020)
8 M. Vukomanovic, S. Skapin, D. Suvorov, EP2863751A1, P-201200204
9 M. Vukomanović et al. Sci. Rep. (Nature Springer). 7 (2017) 4324. ARTICLE DOWNLOAD
10 M. Vukomanovic et al. Colloids and Surfaces B: Biointerfaces, 87, 2011, 226-235. ARTICLE DOWNLOAD
11 M. Vukomanović et al. J. Bionanotechnol. (Nature Springer). (2019) ARTICLE DOWNLOAD
12 M. Vukomanović et al. Small (Wiley –VCH). 2018, 1800205. ARTICLE DOWNLOAD
13 Vukomanović, M. et al. ACS Biomater. Sci. Eng. 1, 935–946 (2015). ARTICLE DOWNLOAD
14 Henkel, J. et al. Bone Res. 1, 216–248 (2013).
15 World Health Organization. Ageing and health. Available at: http://www.who.int/mediacentre/factsheets/fs310/en/ .
16 Murthy, S. E., Dubin, A. E. & Patapoutian, A. Nat. Rev. Mol. Cell Biol. (2017). doi:10.1038/nrm.2017.92
17 Jeremy S. Treger, Michael F. Priest, Raymond Iezzi, and Francisco Bezanilla, Real-Time Imaging of Electrical Signals with an Infrared FDA-Approved Dye, Biophysical Journal Volume 107 September 2014 L09–L012.
18 Easwaran Arunkumar, Christopher C. Forbes, Bruce C. Noll, and Bradley D. Smith, Squaraine-Derived Rotaxanes: Sterically Protected Fluorescent Near-IR Dyes, J. Am. Chem. Soc. 2005, 127, 3288-3289